OVERVIEW
This study focuses on two key players: The Fraxinus spp. (Ash) and Agrilus planipennis a.k.a the Emerald Ash Borer (EAB).
FRAXINUS/ASH
You have probably encountered an Ash wood product in your lifetime. From furniture like staircases, flooring and tables, to tools such as bows, drums shells and baseball bats, versatility is the way of the Ash.
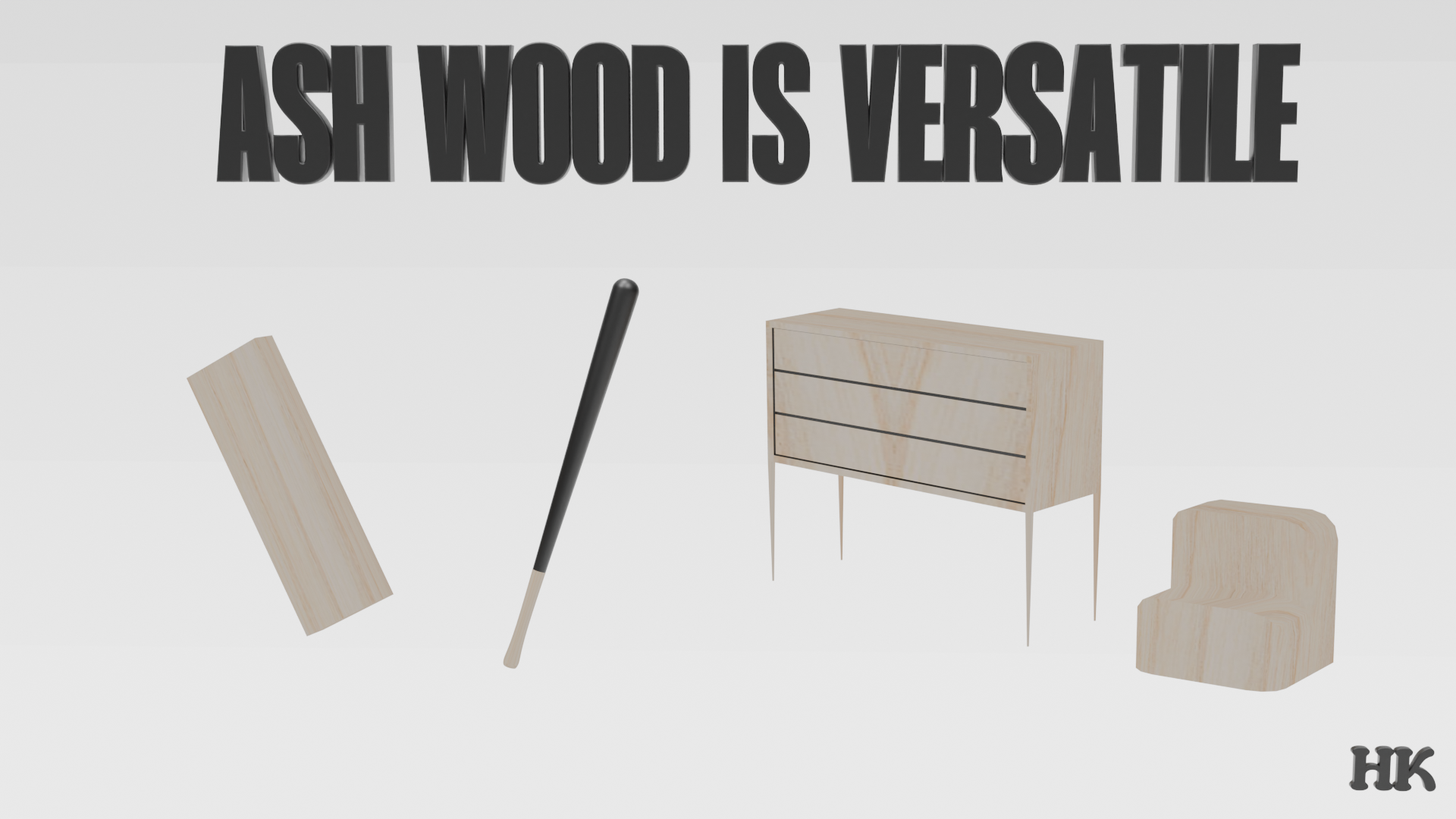
If you enjoy instruments, you’ve probably heard of the brand Fender. You may be interested to learn that Fender exclusively used Ash tone wood for their bass and electric guitar bodies back in the 50s. These guitars are often highly priced and sought after.
While Ash wood produces plentiful products (nice sounding ones at that), the Ash trees themselves are great protectors. Farmers and inhabitants of the coastal regions in China plant Ash trees as windbreaks, and even as decorations and ornaments (Wang et al. 2010) as these trees are tolerant to saline-alkaline soils common to the area (Zhu et al. 2006).
Beyond human society, various Fraxinus species provide key ecological services.
Birds and various mammals nest and feed on the foliage of the Ash trees (Hilty, n.d.). Ash trees also house a myriad of insects from Tropidosteptes, aphids, caterpillars, gall fly larvae, etc. The leaves of Ash trees native to North America are low in tannins, thus serving as a vital resource for frogs and its tadpoles (Stephens et al. 2013). In essence, Ash trees are quite remarkable.

AGRILUS PLANIPENNIS/EMERALD ASH BORER
These tiny wood boring beetles sport a brilliant green, metallic hue. Their size and elegance provide a much tamer impression than they should. The Emerald Ash Borers (EAB) can be absolutely devastating.
As their name suggests, they bore out of Ash trees as part of their life cycle. As larvae, they chew through the bark into the phloem and cambium to nourish itself and develop. This interaction leaves S-shaped galleries which inhibit nutrient and water transportation within the tree, killing it within 2-3 years of infestation (Liu et al. 2003).

Once their development is finished, Adult beetles bore out of their home ash trees to find a mate, leaving behind D-shaped holes on the bark (Gould et al., 2019). These guys are not buddies with the Ash.
Unfortunately, due to the parasitic nature of this organism, even the mighty North American Ash trees fall. 99% of Ash trees are dead 8-10 years after an EAB population is established.
These beetles were first detected in 2002 through infested shipments, but were thought to have landed on American soil since the 90s (Haack et al., 2002). From there on, the EAB killed millions and infested more Ash trees in North America from its establishment in the early 2000s (Matsoukis, n.d.). Interesting enough, they weren’t a threat in the native regions of China, Korea, Japan, Mongolia, Taiwan, and Russia (Haack et al., 2002 & Wei et al., 2004). In fact, an experiment where North American Fraxinus trees were planted in Asia deduced that the North American variants suffer from higher susceptibility to infestations than their Asian counterparts. (Liu et al., 2003 & Rebek et al., 2008).
The variations between the Asian Fraxinus and the North American Fraxinus raised my eyebrows. Perhaps the Asian counterparts had more time and experience dealing with these EABs and have developed some strategies that haven't reached the western hemisphere, but this is all speculation. This research is specifically concerned with Canadian (Southern Ontarian) Ash Trees. These trees are unfortunately suffering a similar fate to their southern American relatives and aren’t handling the invasion well.
LOCATION
Location: 115 A parcel, Lower Lions Club Rd Hamilton, ON
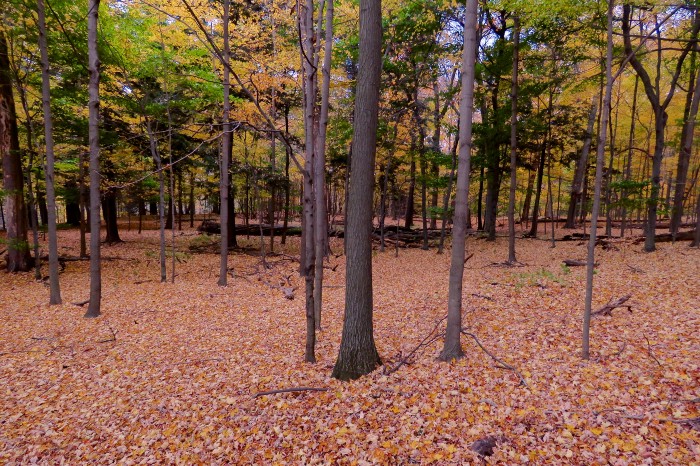
The site of this study is the McMaster Forest situated in Hamilton, Southern Ontario. Spanning 48 hectares within the Carolinian zone (43°25’W, -79°95’N). McMaster forest sits between two nature reserves in the Dundas Valley and Cootes Paradise and is surrounded by densely populated human settlements. The forest experiences a humid continental climate having four distinct seasons and significant temperature variations between summer and winter (Belda et al., 2014). Temperatures in the region fluctuate from a daily average of -4.7°C in January to 22.0°C in July (Environment Canada, 2017).
The area has a history of farming and was previously inhabited by Indigenous people. McMaster University purchased the land in 1964 and 1969 with the intention of developing the land, but later found factors that complicated any plans for construction. The area was named “McMaster Forest” in 2015 and is currently a protected area used for research and recreation.
McMaster Forest houses over 1000 species and is rich with many types of forests. These forests vary in species distribution, tree abundance, and the abiotic factors that influence the ecosystem. Based on those factors, Ecological Land Classifications (ELC) were determined and are used to describe the various forest types within McMaster Forest
The McMaster Forest has been experiencing an invasion from parasites that originated from Asia. 60% of the Ash trees found in the forest have been killed by the infestation of Emerald Ash Borers (Maloney, 2018). Canopy gaps in forests are a natural occurrence, but the consistent clearing from the Emerald Ash Borers have resulted in unprecedented amounts of canopy gaps (Perry, 2018).
COMMUNITY ECOLOGY
Ecologists define communities as a group of organisms that occupy the same place at the same time. The organisms within communities interact with the environment and on multiple different scales to form the components and characteristics of the biological community. Community ecology covers the patterns and interactions at the community level to better inform our understanding of ecology, the environment, and its inhabitants.
OBJECTIVE & HYPOTHESIS
The study aims to update the census and information on the state of EAB infestation at McMaster Forest and examines the inter-species interaction between EAB and Fraxinus to determine the relationship between forest community structure and EAB infestation.
Investigation: Can we predict the EAB infestation rate and intensity through the forest composition and community structure?
Hypothesis:
- Higher Frax. density increases A. planipennis infestation rate
- Higher tree diversity decreases A. planipennis infestation rate
- Higher Frax. density decreases Frax. infestation severity
- Higher tree diversity increases Frax. infestation severity
METHODOLOGY
In order to investigate this big question, I conducted sampling, winter field work, tree census, GIS, R data analysis & visualization. I broke down the experiment into 4 steps:
- Digitizing the McMaster Forest Census on ArcGIS Pro
- Sampling 24 suitable sites/quadrats
- Survey Ash Trees in Selected Quadrats
- Data Analysis
1a. Past Tree Census
20 hectares (500 m x 400 m) were surveyed within the McMaster Forest using the Smithsonian Center for Tropical Forest Science’s (CTFS) technique for censusing large forest plots. Stakes were placed in 20m intervals north to south and east to west with known coordinates to create a grid. The site was partitioned into 100m2 grids consisting of 20 m2 quadrats. Ropes were used to connect the stakes and establish the 20m2 grids. Temporary stakes were placed along 5m intervals N/S and E/W along the 20m grids to divide the 20m2 quadrats to 16 5m2 sub-quadrats.
1b. Digitizing on ArcGIS Pro
Files Used:
The process used four files:
1. Stakes.shp, which contained survey stakes forming the tree plot grid with easting, northing, and stake/quadrat numbers
2. Offsetjointable.dbf, which provided offsets for coordinates based on the SubQ
3. Mainstem Data Sheet (.csv), saved as a .csv without spaces or special characters in file paths or field name
4. Mapping XY Coordinate Data Sheet (.csv), also saved as a .csv with similar formatting rules.
Processing Done:
The .
csv
files were converted to .dbf
format using the Table to Table tool, retaining only essential fields: quadrat
, date
, subQ
, Tag
, x
, and y
. MappingXY
.
dbf
was joined with stakes.shp
based on quadrat
and stakenumber
fields to add coordinate information, and the joined data was exported as xydatejoined.dbf
. New fields (xoffsetadd
, yoffsetadd
, NewX
, NewY
) were added to the attribute table, with type set to “float,” precision to 18
, and scale to 9
. Next, mainstem.dbf
was joined to xydatejoined.dbf
using the Tag_
field, and the result was exported. Then, offsetjointable.dbf
was joined to the most recent .dbf
using the SubQ
field, and this data was exported as the final .dbf
. The field calculator was used to compute new coordinates (xoffsetadded
, yoffsetadded
, NewX
, NewY
), and unnecessary fields were removed.
The final shapefile was created to display tree occurrences with calculated coordinates (NewX
, NewY
) and included all necessary joined and processed attribute data. This was achieved by displaying XY data from the final .dbf
using NewX
and NewY
, and exporting the result as a shapefile.
The 21900 trees were mapped on ArcGIS Pro to create the image below.

2. Site Sampling
Eligible sites to sample were scoped out. Areas that are difficult to access/have no trees of interest (Frax.) are outlined red. The remaining grids are areas that were chosen for sampling. Out of the eligible quadrats, the Gray Dogwood Deciduous Shrub Thicket Type, Dry – Fresh Sugar Maple Oak Deciduous Forest Type areas, and Dry – Fresh Black Walnut Deciduous Woodland Type were the ELCs found within. 24 random samples were obtained stratified to the 3 different ELCs. 8 samples were obtained per ELC with each square considered a sample.
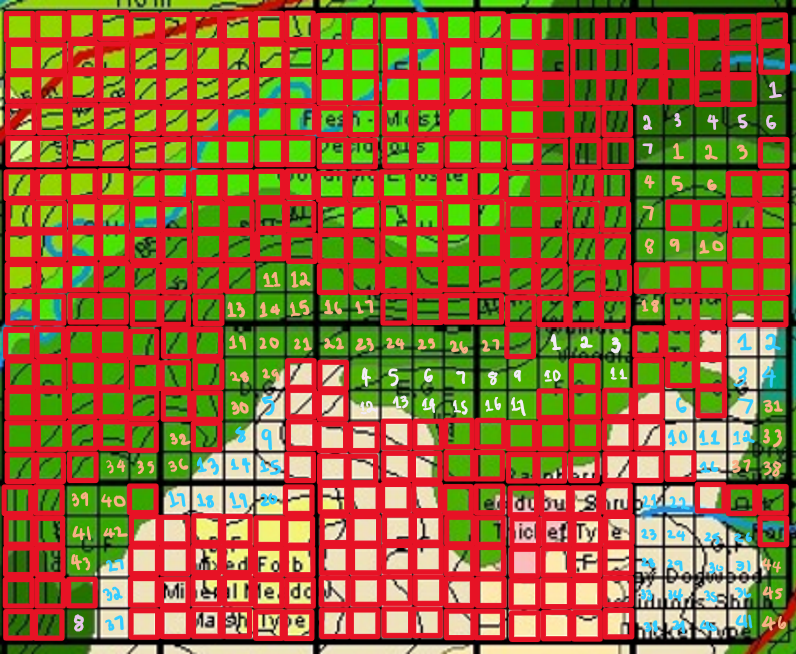
The suitable 400 m2 grids are systematically numbered using whole numbers starting with #1. The quadrats are numbered from left to right then onto the next row where the pattern is repeated until all the quadrats are marked. A random table was generated online with 50 random numbers, a minimum value of 00, a maximum value of 50, and the enabling of duplicate entries.

Each selected 20 x 20 m quadrat were surveyed to determine the condition of all the Fraxinus spp. including their DBH, height of the lowest exit holes, visual assessment of tree condition, and tree location.
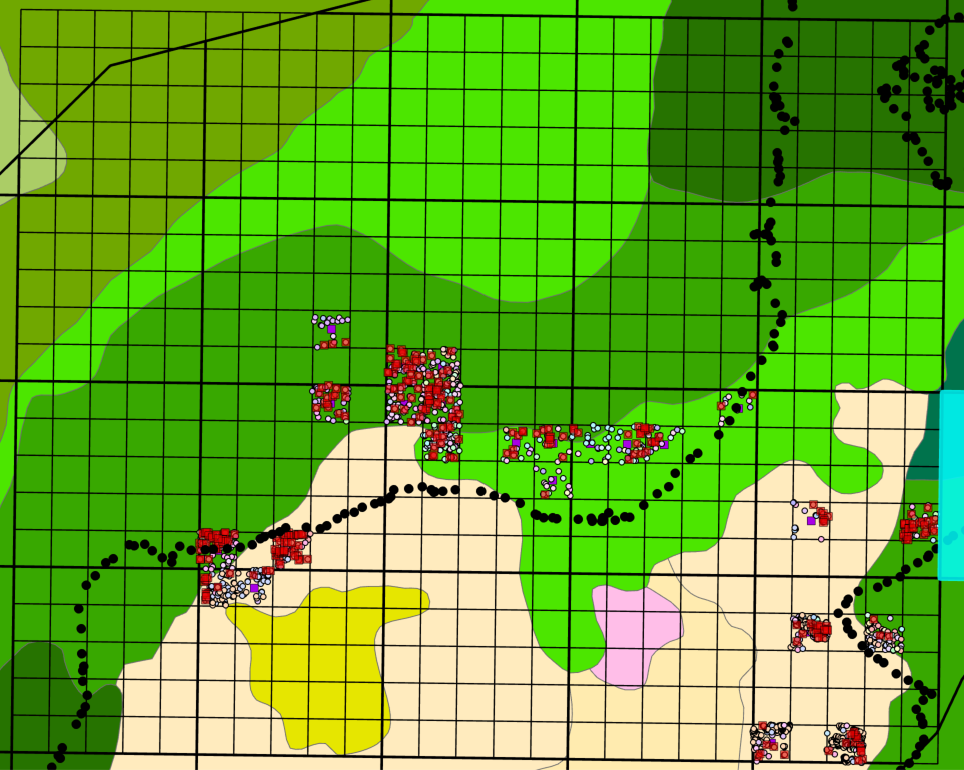
3. Ash Tree Survey
Data collection started during the Winter of 2021 and continued until early Spring of 2022. The 24 selected quadrats add up to 9600 m2 or 1 hectare which makes up 2% of McMaster Forest. Trees that were not discovered in the area were considered dead.
The DBH was obtained by measuring the circumference of the tree 130 m from the base then dividing those values with π on Excel after. Infestation intensity can be estimated by measuring the height of the lowest exit hole from the base of the tree as Agrilus planipennis initially targets the canopy, making its way down the tree as infestation gets more turbulent (Timms et
al. 2006). If the lowest exit hole is higher than 2 m, the tree was considered to either not be infested or be in the early stages of infestation. Each Fraxinus spp. were visually assessed to identify features such as epicormic shoots, lichen cover, peeling bark, larval galleries, and other interesting phenomena. All dead Ash trees were recorded and considered in the analysis to represent infestation intensity.
The entire survey was conducted during winter, which mean I had to identify trees using solely the bark.
4. Data Management
The data obtained from the previous McMaster Forest CTFS census and the tree survey for this study are used to calculate the predictor and outcome variables. The predictor variables include density and diversity metrics per quadrat. The outcome variables include infestation rate, mortality rate, infestation severity and growth rate averaged per quadrat.
Density is calculated using traditional forest community metrics (Grieg, 1983). Density = # of trees sampled of a specific species / area of sample site. The density of all the trees and all the Frax. were calculated to get Tree Density and Frax. Density values per individual 400 m2 quadrat.
There are different indices used to quantify biodiversity. While it is difficult to encapsulate the intricacies of biodiversity with one metric, there are more appropriate indices based on the goal of the analysis. The Shannon index accounts for species richness and evenness while the Simpson’s index can be used to measure species dominance. Morris et al. (2014) found that the Simpson’s Diversity index is most effective at differentiating sites. The Shannon index was deemed appropriate if the rare and abundant species are important in the analysis. Both Shannon and Simpson’s Diversity indices were considered to capture different aspects of biodiversity per quadrat.
Shannon’s Diversity Index was calculated on R based on this equation: Shannon Diversity Index =-Σ[(pi) ∗ log(pi)]. “Σ” is the summation notation. “pi” represents the proportion of individuals present in the i-th species and is solved by: pi = n/N, where n is the sample size of the specific species and N represents the total number of species in the population. Simpson’s Diversity Index was also calculated on R based on this equation: Simpson's Diversity Index = Σ(ni ∗ (ni - 1))/ (N(N - 1)). “ni” is the sample size of a particular species while “N” represents all the individuals in the population.
Agrilus Planipennis Infestation is quantified by the severity and rate. The infestation rate of each 400 m2 quadrat is calculated by: # of infested Fraxinus spp. on site / total # of Fraxinus spp. on site. Agrilus Planipennis infestation severity is set as the average height of the lowest incidence hole per quadrat where lower values indicate more severe cases. The reciprocal value of the average height of the Incidence hole per quadrat was obtained and multiplied by 100 to make the graphs more intuitive. Infestation Severity = (1/(Average Height of Incidence Hole)) ∗ 100. This equation makes higher Infestation Severity values imply more severe infestation.
The growth rate is calculated by the absolute value of the change in values between the previously obtained DBH values and the more current DBH values. The tables below show the individual raw data and the derived values.
The relationship of Fraxinus spp. density and tree diversity (predictor variables) to the infestation rate, average mortality rate and height (outcome variables) were tested through simple linear regressions. The factors in the analysis represents that of the entire 20 x 20 m quadrat. 189 trees within 16 different quadrats were considered in the analysis. 123 of the 189 had complete information with the DBH and lowest emergence hole height values. The small sample size of the analyzed data should be considered when drawing conclusions. Linear regressions and residual analysis were performed on R. The differences in the outcome variables between different ELCs were obtained using ANOVA.
REFERENCES
- Barnes, B.V. (1976). Succession in deciduous swamp communities of southern Michigan formerly dominated by American elm. Canadian Journal of Botany, 54: 19–24
- Belda, M., Holtanová, E., Halenka, T., & Kalvová, J. (2014). Climate classification revisited: From köppen to trewartha. Climate Research, 59(1), 1–13. https://doi.org/10.3354/cr01204
- Cardinale, B., Duffy, J., Gonzalez, A., Hooper, D., Perrings, C., Venail, P., Narwani, A., Mace, G., Tilman, D., Wardle, D., Kinzig, A., Daily, G., Loreau, M., Grace, J., Larigauderie, A., Srivastava, D. and Naeem, S. (2012). Biodiversity loss and its impact on humanity. Nature, 486(7401), pp.59-67.
- Crook, D. J., & Mastro, V. C. (2010). Chemical ecology of the Emerald Ash Borer Agrilus planipennis. Journal of Chemical Ecology, 36(1), 101–112. https://doi.org/10.1007/s10886-009-9738-x
- de Meeûs, T., & Renaud, F. (2002). Parasites within the new phylogeny of eukaryotes. Trends in Parasitology, 18(6), 247–251. https://doi.org/10.1016/s1471-4922(02)02269-9
- Environment Canada. (2017). Meteorological Service of Canada. Canadian Climate Normals. 1981-2010 Climate Normals & Averages. Retrieved from http://climate.weather.gc.ca/climate_normals/index_e.html
- Gandhi, K.J.K. and Herms, D.A. (2010). Potential biodiversity loss due to impending devastation of the North American genus Fraxinus by the exotic emerald ash borer. Biological Invasions, 12: 1839–1846
- Gandhi, K.J.K., Smith, A., Hartzler, D.M., and Herms, D.A.( 2014). Indirect effects of emerald ash borer-induced ash mortality and gap formation on epigaeic beetles. Environmental Entomology, 43: 546–555.Greig-Smith, P. (1983). Quantitative Plant Ecology. Blackwell.
- Gilbert, G., Howard, E., Ayala-Orozco, B., Bonilla-Moheno, M., Cummings, J., Langridge, S., Parker, I., Pasari, J., Schweizer, D. and Swope, S. (2010). Beyond the tropics: forest structure in a temperate forest mapped plot. Journal of Vegetation Science, 21(2), 388– 405.
- Gould, J. S., Bauer, L. S., Duan, J., & Petrice, T. (2019). Emerald Ash Borer, Biological Control Release and Recovery Guidelines. U.S. Dept. of Agriculture, Animal Plant Health Inspection Service, Forest Service Northern Research Station, and Agricultural Research Service.
- Haack, R. A., Jendak, E., Liu, H., Marchant, K. R., Petrice, T. R., Poland, T. M., & Ye, H. (2002). The emerald ash borer: a new exotic pest in North America, 47(3 & 4 ), 1–5.
- Herms, D. A., & McCullough, D. G. (2014). Emerald Ash Borer invasion of North America: History, biology, ecology, impacts, and management. Annual Review of Entomology, 59(1), 13–30. https://doi.org/10.1146/annurev-ento-011613-162051
- Hilty, J. (n.d.). Trees, Shrubs, and Woody Vines of Illinois. Illinois Wildflowers. Retrieved December 27, 2021, from http://www.illinoiswildflowers.info/index.htm
- Kuris, A. M., Hechinger, R. F., Shaw, J. C., Whitney, K. L., Aguirre-Macedo, L., Boch, C. A., Dobson, A. P., Dunham, E. J., Fredensborg, B. L., Huspeni, T. C., Lorda, J., Mababa, L., Mancini, F. T., Mora, A. B., Pickering, M., Talhouk, N. L., Torchin, M. E., & Lafferty, K. D. (2008). Ecosystem energetic implications of parasite and free-living biomass in three estuaries. Nature, 454(7203), 515–518. https://doi.org/10.1038/nature06970
- Liu, H., Bauer, L. S., Gao, R., Zhao, T., Petrice, T. R., & Haack, R. A. (2003). Exploratory survey for the emerald ash borer, Agrilus planipennis (Coleoptera: Buprestidae), and its natural enemies in China. The Great Lakes Entomologist, 36(3 & 4), 11.
- Maloney, J., & Harvey, C. (2018).Analysis of Forest Community Structure Changes as a Result of Non-indigenous Herbivory in an Eastern North American Temperate Forest. McMaster University, Hamilton, ON, Canada.
- Matsoukis, C. (n.d.). Emerald Ash Borer Information Network. Emerald Ash Borer. Retrieved December 27, 2021, from http://www.emeraldashborer.info/
- McCullough, D. G., & Katovich, S. A. (2004). Emerald ash borer pest alert - forest pests. Pest Alert. Retrieved December 27, 2021, from https://www.forestpests.org/acrobat/eab.pdf
- Mitchell, C. E. (2003). Trophic control of grassland production and biomass by pathogens.
Ecology Letters, 6(2), 147–155. https://doi.org/10.1046/j.1461-0248.2003.00408.x - Morris, E. K., Caruso, T., Buscot, F., Fischer, M., Hancock, C., Maier, T. S., Meiners, T., Müller, C., Obermaier, E., Prati, D., Socher, S. A., Sonnemann, I., Wäschke, N., Wubet, T., Wurst, S., & Rillig, M. C. (2014). Choosing and using diversity indices: Insights for ecological applications from the German biodiversity exploratories. Ecology and Evolution, 4(18), 3514–3524. https://doi.org/10.1002/ece3.1155
- Muñoz, S. (2016). Variation in tree and shrub diversity across space, along environmental gradients and through time in a temperate forest in eastern North America. McMaster University, Hamilton, ON, Canada
- Parsons, G. L. (2008, November). Emerald Ash Borer - A guide to identification and comparison to similar species. Retrieved December 28, 2021, from http://www.emeraldashborer.info/documents/eab_id_guide.pdf
- Perry, K. I. (2018, January 4). After Emerald Ash Borer, Invertebrates On the Ground Get to Work. Entomology Today. Retrieved January 29, 2021, from https://entomologytoday.org/2017/12/21/after-emerald-ash-borer-comes-and-goes- invertebrates-on-the-ground-get-to-work/
- Preston, D. L., & Johnson, P. T. J. (2010). Ecological Consequences of Parasitism. the nature education. Retrieved December 29, 2021, from https://www.nature.com/scitable/knowledge/library/ecological-consequences-of- parasitism-13255694/
- Polis, G. A., & Hurd, S. D. (1996). Linking marine and terrestrial food webs: Allochthonous input from the ocean supports high secondary productivity on small islands and coastal land communities. The American Naturalist, 147(3), 396–423. https://doi.org/10.1086/285858
- Rebek, E. J., Herms, D. A., & Smitley, D. R. (2008). Interspecific variation in resistance to emerald ash borer (Coleoptera: Buprestidae) among North American and Asian ash (fraxinus spp..). Environmental Entomology, 37(1), 242–246. https://doi.org/10.1603/0046- 225x(2008)37[242:ivirte]2.0.co;2
- Simone, K., & Harvey, C. (2017). Emerald Ash Borer at McMaster Forest. McMaster University, Hamilton, ON, Canada.
- Stephens, J. P., Berven, K. A., & Tiegs, S. D. (2013). Anthropogenic changes to leaf litter input affect the fitness of a larval amphibian. Freshwater Biology, 58(8), 1631–1646. https://doi.org/10.1111/fwb.12155
- Stevens, A. N. P. (2010). Predation, herbivory, and parasitism coexist within ecological communities. How do these interactions create dynamic, ever-changing biological systems? the nature education. Retrieved January 29, 2021, from https://www.nature.com/scitable/knowledge/library/predation-herbivory-and- parasitism-13261134/
- Timms, L. L., Smith, S. M., & de Groot, P. (2006). Patterns in the within-tree distribution of the Emerald Ash Borer Agrilus planipennis (Fairmaire) in young, green-ash plantations of south-Western Ontario, Canada. Agricultural and Forest Entomology, 8(4), 313–321. https://doi.org/10.1111/j.1461-9563.2006.00311.x
- Wang, X.-Y., Yang, Z.-Q., Gould, J. R., Zhang, Y.-N., Liu, G.-J., & Liu, E. S. (2010). The biology and ecology of the emerald ash borer,Agrilus planipennis, in China. Journal of Insect Science, 10(128), 1–23. https://doi.org/10.1673/031.010.12801
- Wei, X., Reardon, D., Wu, Y., & Sun, J. H. (2004). Emerald ash borer, Agrilus planipennis Fairmaire (Coleoptera: Buprestidae), in China: a review and distribution survey. Acta Entomologica Sinica, 47(5), 679-685.
- Zhu, G. H., Liu, L., Soreng, R. J., & Olonova, M. V. (2006). Poa L. Flora of China, 22, 257-309.