OVERVIEW
"Whatever you put into the ground has to end up somewhere"
LEACHING
Agricultural leaching refers to the process where water-soluble substances, such as nutrients or pesticides, are carried away from the soil by water movement. Leaching can occur naturally through rainfall or irrigation, carrying solutes like nitrates and phosphates into nearby water bodies or deeper into groundwater aquifers. This seemingly innocuous phenomenon has profound implications for ecosystems and human health. When fertilizers dissolve and percolate through the soil, they don’t simply disappear.
Among the soil nutrients, nitrogen is leached the most with 16% being lost via leaching and only 55% of the N output being used by plants (Liu et al. 2010). When nitrogen is leached not only is the environment negatively impacted, but so is the soil fertility and crop production (Matson 1997).
The importance of understanding and mitigating leaching cannot be overstated. Excess nutrients leached from agricultural fields often find their way into rivers, lakes, and oceans, causing eutrophication – an overabundance of nutrients in water bodies. This process triggers algal blooms, depletes oxygen levels, and creates dead zones where aquatic life struggles to survive.
Beyond surface water, leaching can also contaminate groundwater aquifers. These underground water sources, relied upon for drinking water and irrigation, are especially vulnerable to pollution from water-soluble pesticides and nitrates. The result is a ripple effect: ecosystems that provide invaluable services, from habitats for biodiversity to resources for industries, agriculture, and tourism, are compromised.
MYCORRHIZAE
Amid this challenge lies an unlikely ally: mycorrhizal fungi. Having spent three years (as of 2021) working at the Royal Botanical Gardens and the McMaster Biology Greenhouse, I’ve become an avid gardener. In my pursuit of the perfect substrate for my plants, I frequently encountered the buzzword “mycorrhizae.” Eventually I saw it so much that I had to look it up.
To my amazement, I discovered that mycorrhizal fungi form symbiotic relationships with over 95% of terrestrial plants. These fungi extend the reach of plant roots through their intricate networks of hyphae, which act as natural conduits for water and nutrients. In return, plants supply the fungi with carbohydrates. This system is so widely adopted that plants without these fungal partners are the odd ones out.
Plant-mycorrhizal symbiosis has potential use in bioremediation and restoration of environments. Vegetation contributes a huge role in increasing the moisture retained on land. Surface runoff is greater in bare land compared to well vegetated areas (Pielke and Niyogi 2010). The plant’s function in exchanging carbon dioxide and water vapor with the atmosphere makes it an important component of the hydrological cycle. Arbuscular mycorrhizal fungi (AMF) tend to form symbiosis with compatible plant species. The AMF colonize the cortical cells of the plant host’s roots to access the plant carbons. The mycorrhizae improve the plant’s nutrient uptake and resistance towards stressors in exchange for the plant’s carbon. AMF fungi were found to reduce N leaching and improve plant P contents (Bender et al. 2015 & Tran et al. 2020).
OBJECTIVE
The aim of this project is to explore the effects of plant-mycorrhizal symbiosis on the environment. The ability of mycorrhizae to extract nutrients from the soil and make it available to plants seem to be related to the concentrations of nutrients that enter the water bodies. The approach to determining whether there is a relationship between mycorrhizae and leeching is to create a miniature model and imitate the abiotic and biotic features involved. Mycorrhizae’s environmental role should be further explored to maximize their use and benefits in restoration.
METHODOLOGY
The experiment can be divided into 5 steps:
- Obtaining the seeds + Fieldwork to collect riparian seeds
- Prepping the substrate
- Growing plants in greenhouse
- Designing + Building miniature farm + waterbody models
- Measuring the Nitrogen
1. Obtaining the Seeds
The Farm is divided into a crop and riparian zone. The crop zone contains typical agricultural crops, and will be the area that is fertilized. The riparian zone is the buffer area between the farm and the waterbody, and contains rich and moist soils that can house diverse plant communities.
Corn (Zea mays) seeds were chosen to be used as the primary crop zone plant. The seeds were obtained from the Native Seed SEARCH from Tucson AZ.
The riparian zone plants were obtained out in the wild. The reason is to get plants that are naturally in a riparian zone. Sampling was conducted in the Lot M field in Hamilton in February 2021. Lot M is near Spencer Creek and is situated in between Cootes Paradise and the Dundas Valley Conservation Area. The seeds were harvested from the adult plants found in the sampled plots. The species include: Rudbeckia hirta (Black-eyed Susan), Solidago (Goldenrod), and Elymus canadensis (Canada wildrye).
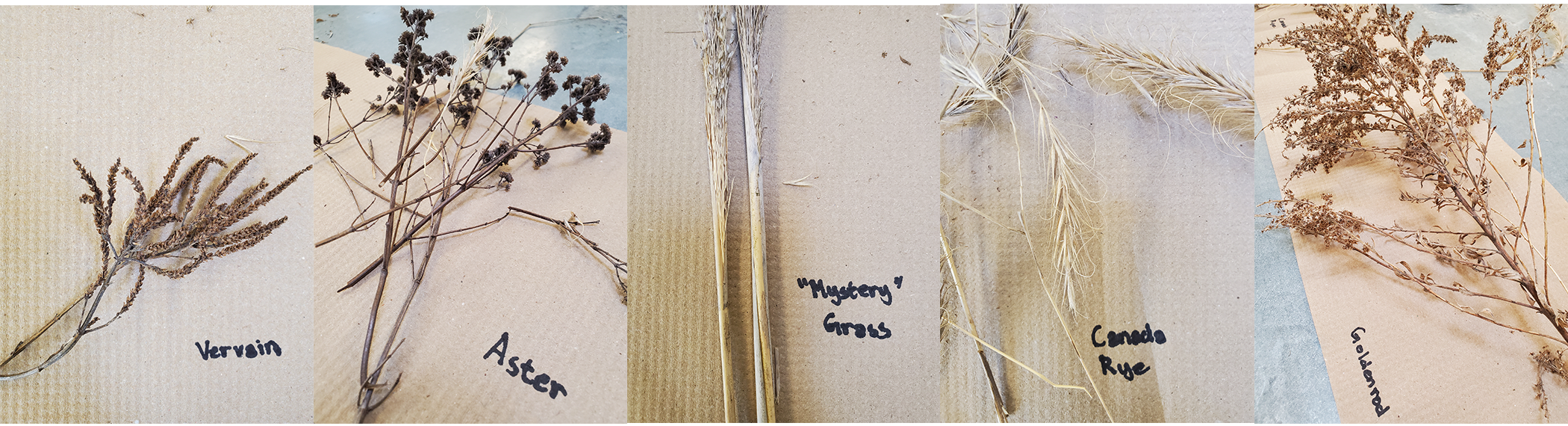

2. Prepping the Substrate
PRO-MIX HP was used as the substrate. The mix contains sphagnum peat moss, perlite, limestone, and a wetting agent. The mycorrhizae found in the substrate is Rhizophagus irregularis (PTB297 Technology). Mycorrhizae treatment groups used the PRO-MIX HP., while the groups without mycorrhizae used autoclaved PRO-MIX HP. The substrate was autoclaved for 4 cycles (30 min per cycle) at 121 degrees Celsius % 15 – 20 psi to remove the mycorrhizae fungi (and any other organisms) within.
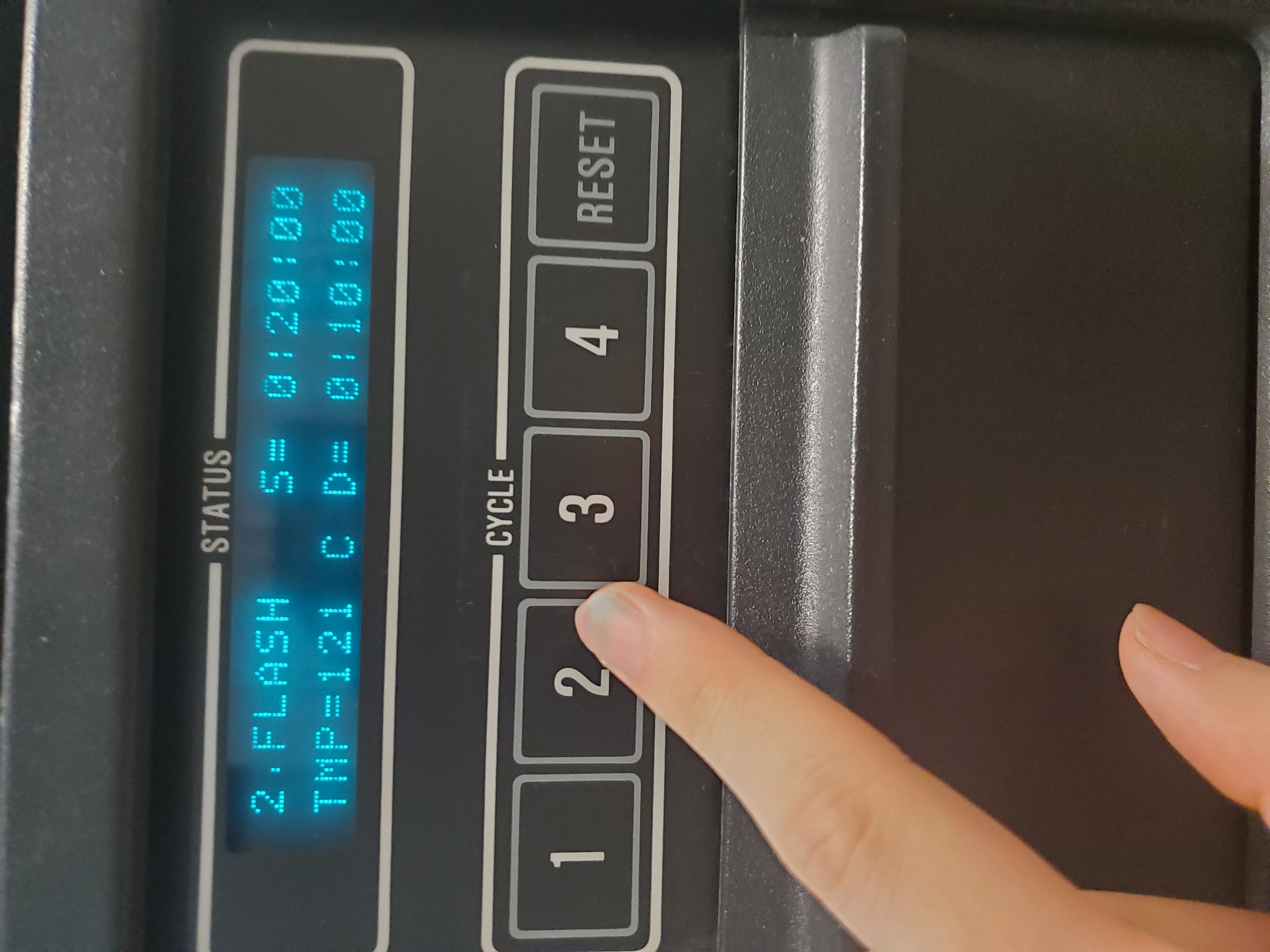
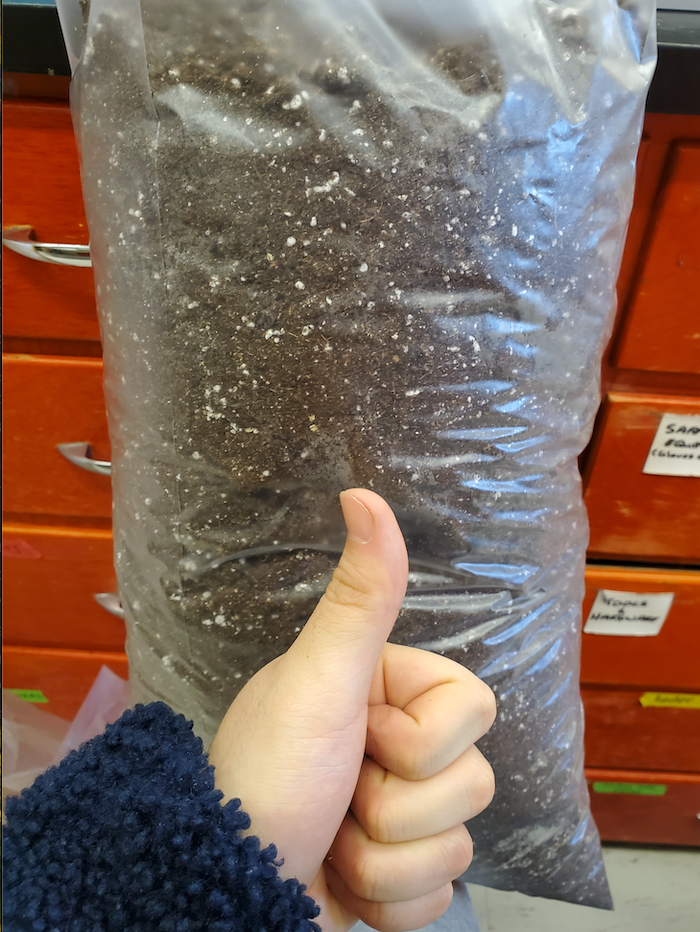
3. Growing the Plants
Plants were germinated and developed from February to April in the McMaster Biology Greenhouse. The groups were already separated between mycorrhizal and non-mycorrhizal. The plants in the mycorrhizal group are grown in the aforementioned PROMIX while the non-mycorrhizal group are grown in sterilized soil.
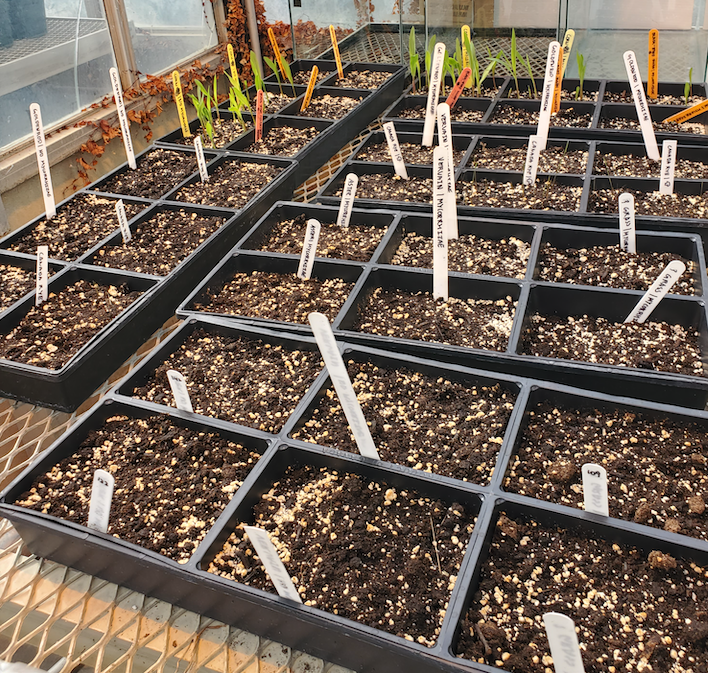
Throughout the experiment, I found that the plants grown in mycorrhyzae had more robust, thick roots while the plants grown in the sterile substrate had weak, long roots. If you don't believe me, see for yourself!


4a. Designing and Building the Farms
For the waterbodies, simple aquariums were used. 6 tanks measuring 20 in (L) x 10.5 in (H) x 12 in (W) were obtained from the McMaster Biology Department.
Making the farm was no easy feat. The total time it took to design, prototype and eventually woodwork all of these tanks took around 2 months. I have put some of the pictures of the journey along the way.
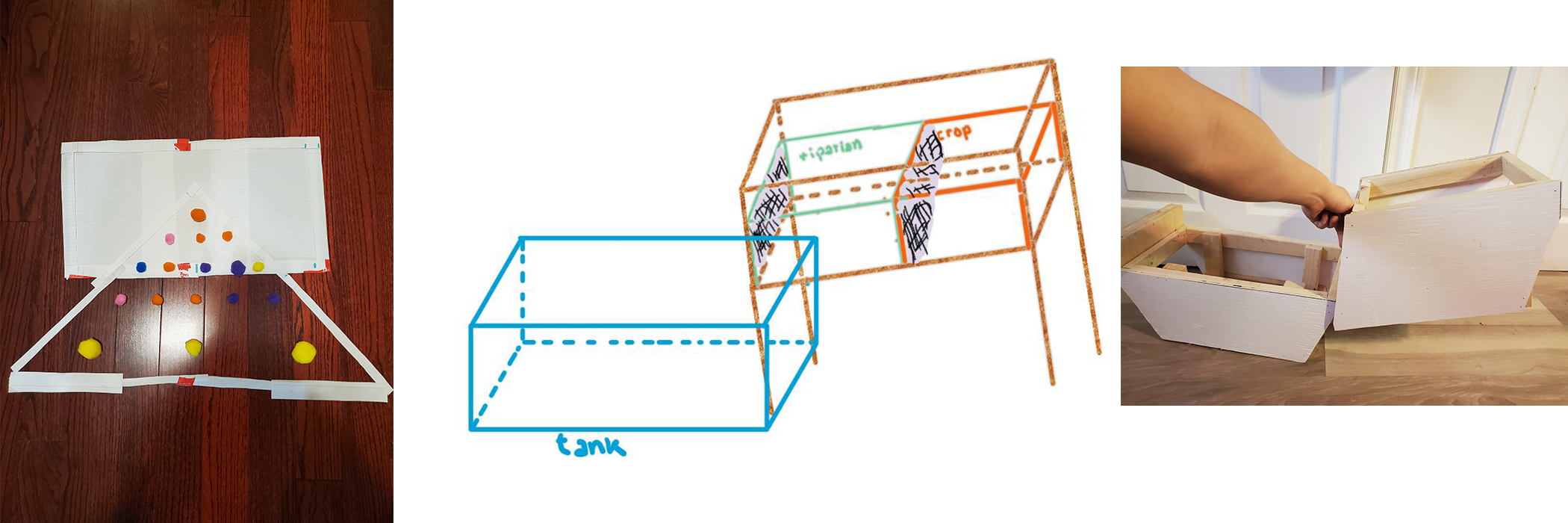


4b. Setting up the Farms
All the mesh and tanks were sterilized accordingly before being used.
The experiment was conducted between April to May 2021. Abiotic factors include fertilizer applications and precipitation. Precipitation is simulated in increments of 1L per tank every 3 days for 2 weeks. A watering can was used in order to control the volume. Watering was done very carefully over the crop and riparian zone to imitate precipitation while minimizing the erosion.
REFERENCES
- Bender SF, Conen F, Van der Heijden MGA. Mycorrhizal effects on nutrient cycling, nutrient leaching and N2O production in experimental grassland. Soil Biology and Biochemistry. 2015;80:283–292. doi:10.1016/j.soilbio.2014.10.016
- Dymond JR. Wetland ecosystem services. In: Ecosystem services in New Zealand. Lincoln, N.Z.: Manaaki Whenua Press; 2013. p. 192–202.
- Dymond JR. Conditions and trends. In: Ecosystem services in New Zealand. Lincoln, N.Z.: Manaaki Whenua Press; 2013. p. 203–225.
- Liu J, You L, Amini M, Obersteiner M, Herrero M, Zehnder AJ, Yang H. A high-resolution assessment on global nitrogen flows in cropland. Proceedings of the National Academy of Sciences. 2010;107(17):8035–8040. doi:10.1073/pnas.0913658107
- Matson PA. Agricultural Intensification and Ecosystem Properties. Science. 1997;277(5325):504–509. doi:10.1126/science.277.5325.504
- NOAA. Water cycle. Water cycle | National Oceanic and Atmospheric Administration. 2019 Feb [accessed 2021 Mar 19]. https://www.noaa.gov/education/resource-collections/freshwater/water-cycle
- O'Callaghan AM. Mycorrhizae. [accessed 2021 Apr 16]. https://www2.nau.edu/~gaud/bio300/mycorrhizae.htm
- Pielke RA, Niyogi D. The Role of Landscape Processes within the Climate System. 2010 [accessed 2021 Mar 18]. https://www.researchgate.net/publication/225660029_The_Role_of_Landscape_Processes_within_the_Climate_System
- Tran CTK, Watts-Williams SJ, Smernik RJ, Cavagnaro TR. Effects of plant roots and arbuscular mycorrhizas on soil phosphorus leaching. Science of The Total Environment. 2020;722:137847. doi:10.1016/j.scitotenv.2020.137847
- Zheng X-K, Li C, Huang G-H, Yang Z-F. Research progress in effects of urbanization on wetland ecosystem in watershed. ResearchGate. 2008 Mar [accessed 2021 Mar 19]. https://www.researchgate.net/publication/289803357_Research_progress_in_effects_of_urbanization_on_wetland_ecosystem_in_watershed